Physics
- Swift E. Creek
- Apr 24, 2017
- 13 min read
PHYSICS
Physics is the study of matter and energy. It is one of humanity’s oldest academic disciplines, and a basic understanding of physics is essential on some level for all of the other sciences.
ENERGY
In physics, work and energy are very closely related topics. Energy can be defined as the ability to do work or to cause change. Work, interestingly enough, can be describe as a change or transfer of energy. So, energy and work are used to define each other. They are even measured using the same unit, Joules (J); a metric unit combining force and distance.
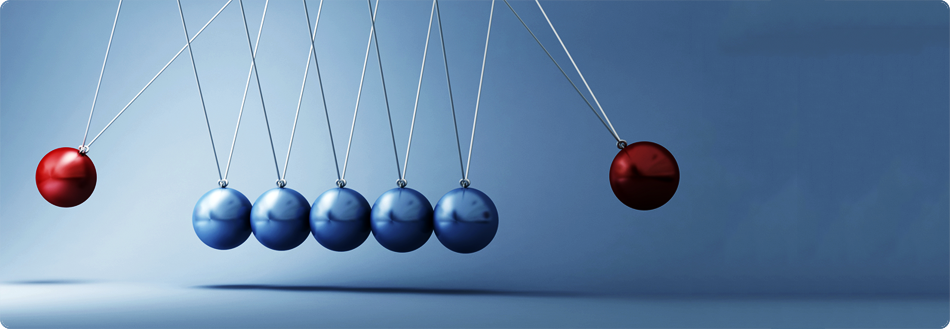
Energy is essential for life, and is the driving force for nearly all of the systems we observe on Earth. In order to understand how energy works and affects our environment, it is important to know about the many forms of energy.
THERMAL ENERGY
All matter is made of atoms; and those atoms are constantly in motion. Either vibrating in place such as in a solid material or flying around excitedly such as in a gas, the atoms that make up all substances have energy that causes them to move. Thermal energy refers to the total energy of the particles in an object. In other words, if you add up the energy of each individual particle in the object, you will have its thermal energy.
Temperature
Temperature is often confused with thermal energy. While thermal energy is the total energy of the particles in an object, temperature is a measurement of the average kinetic energy of the particles in a substance. In other words, when we measure the temperature of something, we are measuring how fast its particles are moving. Higher temperatures indicate that the particles are moving faster while lower temperatures indicate that the particles are moving slower. Objects with the same thermal energy do not necessarily have the same temperature. For example, a very small but hot object such as a match may have the same thermal energy as a very large but cold object such as an ice sculpture. Likewise, objects with the same temperature do not necessarily have the same thermal energy. Two cups with different amounts of room temperature water will have different thermal energy. The cup with more water has more particles, thus more total energy.
The metric unit of measure for temperature is degrees Celsius (°C). The Celsius scale was developed to coincide with the melting point and boiling point for water, a substance most people are very familiar with. 0°C is the temperature that water freezes, and 100°C is the temperature that water boils. In physics and chemistry, we often use another measurement for temperature, Kelvin (K). The Kelvin temperature scale is based on the Celsius scale, but it is shifted down so that 0 K coincides with absolute zero, the theoretical temperature at which there is no particle motion at all. A third common unit of temperature, Fahrenheit (°C), is non-metric and only used in some parts of the world (including the United States).
Heat
Like temperature, many people often confuse the concept of heat with thermal energy. In science, heat refers to the transfer of thermal energy between substances. Heat always flows from warmer substances to cooler substances until they reach the same temperature, called the equilibrium temperature. This is why we feel the sensation of “hot” or “cold” when we touch hot and cold objects. If you sit on a metal slide on a hot summer day, you may burn your legs. This is because the slide is warmer than your skin, so thermal energy flows from the slide into your legs, causing a “hot” sensation. Likewise, if you hold an ice cube in your hand, you will feel a “cold” sensation. This is because your hand is warmer than the ice cube, so thermal energy flows out of your hand into the ice cube. The energy leaving your hand causes you to sense “cold” and can be seen melting the ice cube. It is important to realize that “cold” is simply a sensation. There is no such thing as “cold” or “cold energy”. Things we refer to as cold simply have less thermal energy that what we perceive as a normal, or room temperature.
There are three types of heat: conduction, convection, and radiation. Conduction transfers thermal energy by contact between particles. An example of conduction would be touching a hot stove or placing your hand in a bucket of ice water. Materials that transfer thermal energy easily or quickly are called thermal conductors. Metals, diamonds, and other dense substances tend to be good thermal conductors. Thermal insulators are materials that transfer thermal energy slowly or poorly. These types of substances include paper, wood, or cotton; materials that have a relatively low density.
Convection transfers thermal energy by the movement of currents in a fluid (a liquid or a gas). Convection currents form when a fluid is unevenly heated. Warmer fluid becomes less dense and rises to the top, while cooler fluid becomes denser and sinks toward the bottom. This creates a continuous current as the fluid heats and cools. An ocean breeze often forms in this way, with the warm air over the beach rising and the cool air over the water sinking and blowing on-shore.
Radiation transfers thermal energy through electromagnetic waves (light). Warming up by a campfire or keeping fast food warm under a heat lamp are both examples of radiation. We can also see radiation transferring thermal energy when Earth is warmed by sunlight. This example illustrates a unique aspect of radiation. Unlike conduction and convection, which both require matter to transfer thermal energy, radiation can transfer thermal energy through a vacuum such as space.
CHEMICAL ENERGY
Chemical energy is stored in chemical bonds and released when those bonds are broken. When plants use sunlight to make carbohydrates during photosynthesis, the energy from the sunlight is stored in chemical bonds in the carbohydrates. When animals eat those carbohydrates, their bodies convert the stored energy into ATP which fuels their life functions.
NUCLEAR ENERGY
Energy is also stored in the nuclei of atoms; this energy is called nuclear energy. Nuclear energy can be released during two types of nuclear reactions, fission and fusion. Nuclear fission occurs when nuclei are bombarded with a stream of sub atomic particles which blasts them apart releasing an incredible amount of energy (“fission” means to split). Nuclear fusion occurs when atoms are smashed together under such intense pressure that the nuclei join together, or fuse. This too, releases incredible amounts of energy. Nuclear fission is the type of energy used in nuclear power plants and nuclear weapons. Nuclear fusion is the energy source that powers stars such as our Sun.
ELECTRICAL ENERGY
Energy carried by a flow of electrons is called electrical energy, or electricity. We can see evidence of electrical energy in lightning, electrical appliances, or stored in batteries to power our devices. Some materials, such as copper wire, allow electrons to flow through them easier than others. These materials are called electrical conductors. Substances that impede, or limit, the flow of electrons through them are called electrical insulators. Plastics and rubber are often used for this purpose.
SOUND ENERGY
There are two types of energy that are carried by waves. One of these is sound energy. Sound energy, carried by sound waves, relies on particles bumping into each other to transmit the energy through a medium. Without particles to bump into each other, there can be no sound. This is why there can’t be sound in the vacuum of space.
RADIANT ENERGY
The other type of energy that is carried by waves is called radiant energy. Radiant energy is transferred in the form of electromagnetic waves. Electromagnetic waves consist of oscillating (moving back and forth) electric and magnetic fields, like two waves that travel together perpendicular to each other.

There are many types of electromagnetic waves that make up the electromagnetic spectrum, and they are classified by their wavelength.
Radio waves and microwaves have the longest wavelengths of the electromagnetic spectrum, while x-rays and gamma rays have the shortest wavelengths but the highest energy. In the middle of the electromagnetic spectrum are the three main types of light that we get from the Sun, infrared light, visible light, and ultraviolet light.
Visible light, like its name suggests, is the type of light that humans use to see. It ranges in color from red to violet depending on the wavelength (red being the longest visible wavelength and violet being the shortest). When all of these wavelengths are present together in the light, such as sunlight or a standard light bulb, we call it “white light”. Infrared light is just outside the visible spectrum, with longer wavelengths than red light. It is often associated with thermal radiation, though other wavelengths can be responsible for heat. Ultraviolet light, just outside of the visible spectrum on the violet side, has wavelengths that are shorter than visible light. This type of radiant energy is often associated with sunburns.
Light waves and sound waves both interact with their surroundings in a number of ways. When they encounter a surface or a new medium (substance), waves can be reflected, refracted, or absorbed. If they bounce off the surface, we say the waves are reflected. The law of reflection states that waves will reflect at the same angle with which they hit a surface. If a wave passes into or through a new substance, it will refract, or change its angle of motion (bend). This is because waves travel at different speeds through different mediums, so the light or sound will change direction as it speeds up or slows down depending on the substance. Sometimes, waves are absorbed by a material. When certain wavelengths are absorbed by a substance and others are reflected, it affects the color we see. For example, if red wavelengths are reflected off of a shirt and the other wavelengths are absorbed, our eyes will see the shirt as red.
MECHANICAL ENERGY
Objects that have energy as a result of their motion or position are said to have mechanical energy. There are two types of mechanical energy, kinetic and potential. Kinetic energy is the energy of motion; it is affected by an object’s mass (amount of matter) and velocity (speed & direction). Potential energy is energy that is stored in an object based on its position. Mechanical energy can be stored by stretching or compressing an object such as a rubber band or a spring. This is called elastic potential energy. Energy can also be stored in an object by simply raising it off the ground. This type of potential energy, called gravitational potential energy, depends on the mass of the object and its height off the ground.
The mechanical energy of an object changes as its circumstances change. For example, imagine standing in a second-floor window holding a rubber ball over a sidewalk. As you hold the ball in your hand on the second floor, it has no kinetic energy because it is not moving, but it does have gravitational potential energy because it has height. When you release it and it begins to fall, the mechanical energy in the ball converts from potential energy to kinetic energy. As it loses height, the potential energy decreases; but its kinetic energy increases as it gains speed while it falls. By the time the ball reaches the ground it has no more gravitational potential energy because it no longer has height. However, it is moving its fastest just before it hits the ground, so its kinetic energy is at a maximum at that point. When it hits the ground, it briefly stops moving, so its kinetic energy goes back to zero for an instant. However, it compresses upon contact, so it gains elastic potential energy which causes it to bounce. On its way back up, this process happens in reverse, as kinetic energy decreases while it slows down and gravitational potential energy increases as it goes higher.
CONSERVATION OF ENERGY
Energy Conversions
In the above scenario, we illustrated how energy changes from one type of mechanical energy to another. This is called an energy conversion. Energy is constantly being converted between different forms. For example, when the flow of water is used to power a hydroelectric dam, energy is converted from gravitational potential energy (the water at the top of the dam) to kinetic energy (the water flowing down through the dam & spinning turbines) to electrical energy (the spinning of magnets in a generator creates a flow of electrons) that is sent to your home and converted to radiant energy (if you turn on a light), thermal energy (if you turn on the oven), sound energy (if you turn on the radio), etc…
Law of Conservation of Energy
The law of conservation of energy tells us that energy can be converted between all of the different forms of energy but it cannot be created or destroyed. This means that the amount of energy present at the end of a process will equal the amount of energy present before it began; however, this can sometimes be difficult to see. For example, in the scenario above where you drop a ball from a second-floor window, it can appear as though energy is lost. You might expect that when the ball bounces it should reach the same height that it was dropped from if energy is conserved. After all, if it started with a certain amount of gravitational potential energy, it should have the same amount after its bounce, right? Wrong.
As the ball falls through the air, some of its energy is transferred into the air as thermal energy because of friction with the air particles. When it hits the ground, some of its energy is converted into sound energy as it causes vibrations in the air and ground. There is also friction as the rubber squeezes together, which converts more of the energy in the ball to thermal energy. Of course, as the ball travels back up after its bounce, it is again converting some of its energy into thermal energy because of friction with the air. All of these little energy conversions add up to mean that some of the gravitational potential energy the ball began with has been transferred out of the ball or converted to thermal energy, so it will not have the same amount of gravitational potential energy after the bounce. That is why a ball usually has a lower bounce height than its initial drop height. The energy is not lost or destroyed, only converted or transferred.
FORCES
Earlier, we said that work is a change in energy or a transfer of energy. Work can be done on an object by applying a force to it over a distance. A force is a push or a pull on an object; when one object pushes or pulls another we say that it exerts a force. There are several different types of forces, but they can all be described in terms of their strength and direction.
Types of Forces
Gravity is an attractive force between any two objects with mass. Its strength depends on the amount of mass the objects have and the distance between them. Because the mass of the Earth is so much larger than any other object we encounter on a daily basis, we tend to think of gravity as the force that attracts us to Earth. However, all objects with mass exert a gravitational force on objects near them. Because gravity acts on objects at a distance (without touching them), we classify it as a non-contact force. Magnetism is another example of a non-contact force.
When one object applies a force to another by touching it, we call it a contact force. Throwing a ball or pushing a box would be examples of an applied contact force. Friction, also, would be considered a contact force because it only occurs when two surfaces rub against one another.
Net Force
Objects are often under the influence of multiple forces at any given time. For example, when you throw a ball, the ball is experiencing the push from your hand, the pull of gravity, and the drag of air resistance (friction). If we add these forces together based on their strength and direction, the resulting force is called the net force. When the forces exerted on an object cancel each other out, for example, equal forces in opposite directions, the net force is zero. Imagine a game of tug-o-war where both teams are pulling with the same force; the force in one direction will balance out the force in the opposite direction. If the net force is zero, we say there are balanced forces. However, if one or more forces are stronger than the others, then the net force is non-zero and we say there are unbalanced forces. When an object is experiencing unbalanced forces, the strength and direction of the net force affects the motion of the object.
MOTION
In order to determine if an object is in motion or not, we must use reference points. A reference point can be any other object or place, the relative position of which is compared to the object to see if it is changing over time. For example, when you are riding in a car you may use a tree by the side of the road as a reference point to judge whether you are moving. If your position is changing relative to the tree, you will perceive motion. If your position relative to the tree is not changing, you will conclude that you are motionless. Therefore, motion can be defined as a change in position over time relative to a reference point.
Speed is often used to describe the motion of an object. It is calculated by dividing the total distance an object has travelled by the time it took to travel that distance. Speed can be graphed as a function of distance vs. time, where distance is the y-variable and time is the x-variable. When graphed this way, the slope of the line represents the speed of the object; the steeper the line, the faster the speed.

People often use the term velocity as a synonym for speed, however, it has a different meaning in physics. Velocity is the speed of an object with respect to its direction. So, two objects can be travelling at equal speeds, but if they are headed in different directions they have different velocities.
If the motion of an object is changing, we say that it is accelerating. We tend to think of acceleration as an object speeding up; however, acceleration refers to any change in an objects motion, whether it be speeding up (positive acceleration), slowing down (negative acceleration), or even changing direction.
Newton’s Laws of Motion
In the late 1600’s, Isaac Newton described the relationship between the forces exerted on an object and its motion using three physical laws, known collectively as Newton’s laws of motion. Newton’s first law, often referred to as the principle of inertia, states that an object’s motion will remain constant unless it is acted on by an unbalanced force. This means that an object at rest will remain at rest, and an object in motion will maintain a constant velocity unless an unbalanced force changes its motion. Inertia refers to the tendency of objects to resist a change in their motion. It depends on an object’s mass; the greater the mass, the more inertia it has and the harder it is to change the motion of the object.
Newton’s second law describes how the net force affects the motion of an object. It states that the net force exerted on an object is a product of its mass and its acceleration; in other words, net force equals mass times acceleration (F=ma). Further, it states that the object will accelerate in the same direction as the net force. Newton’s second law can help us understand the relationship between mass and weight. The force that Earth’s gravity exerts on an object is called weight; weight is a force. The object’s mass, the amount of matter contained within it, is a physical property of that object. So, weight is the effect that gravity has on mass; they are not synonyms.
Newton’s third law, often called the action-reaction law, says that when one object exerts a force on another object, then the second object exerts an equal but opposite force on the first. In other words, for every action there is an equal but opposite reaction. As you are sitting in your chair reading this, your weight is exerting a downward force on the chair. You may feel the pressure on your back-side where your body is coming into contact with the chair. The pressure you feel is because the chair is exerting an equal but opposite upward force on your bottom. As long as these two forces balance, you will remain seated. However, if your weight is stronger than the upward force the chair is capable of providing, you will crush the chair and accelerate downward!
Comments