Earth in Space and Time
- Swift E. Creek
- Apr 25, 2017
- 15 min read
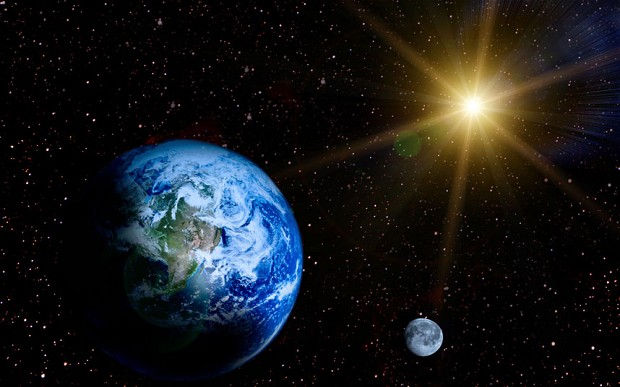
EARTH IN SPACE & TIME
Through the centuries, one of the greatest challenges in science has been understanding Earth’s place in the universe. Even before science became an academic discipline, scholars looked to the heavens and pondered Earth’s interactions with the Sun, Moon, and stars. Since then, written language has allowed our ideas and observations to be recorded for future generations, knowledge has become more accessible, and technology has advanced exponentially. As a result, we’ve been able to look further and further into the universe. Our study of space provides an excellent example of the ever-changing nature of scientific knowledge and the never-ending quest to learn more about our planet and its place in the cosmos. Still, space proves difficult to study because of the distances involved, the dangerous challenges to be overcome, and the financial cost of exploration.
EARTH AND THE SUN
It’s easy to see why the Sun has captured the attention of men since ancient times. By far the closest star to Earth, it is significantly brighter than anything else in the sky. It is so close to us that we can feel its heat. Its energy is what drives the systems on Earth that make life possible. Its rhythmic rising and setting each day provide routine and structure for our lives. The impact that the Sun has on our planet is important for us to explore if we are to understand Earth’s place in the universe.
Earth’s Shape
All objects in the universe with a significant mass have a spherical shape due to the force of gravity. Gravity is a force of attraction between objects with mass. When an object as massive as Earth forms, its own gravitational force, pulling in all directions toward the center of the object, shapes it into a sphere. Like many other objects in our Solar System, Earth spins around a central axis. This spin is called rotation. Earth’s rotation causes a slight bulge around Earth’s center (the Equator), called the equatorial bulge. This means that Earth’s circumference around its equator is slightly larger than its circumference around the poles.
Earth’s Orbit
In addition to influencing Earth’s shape, gravity also causes Earth’s orbit around the Sun. An orbit is the path an object follows as it moves around another object. The motion of an object along this orbital path is called revolution; one full revolution around the Sun is called a year. Earth revolves around the Sun on an elliptical path, with the Sun at one focal point of the ellipse. You can picture an ellipse as an elongated circle with two focal points in the middle instead of one at the center.
Seasons

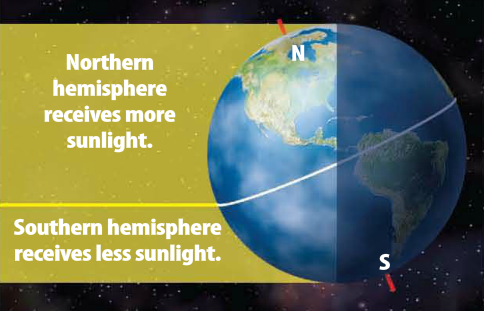
Most places on Earth experience distinct seasons: winter, spring, summer, and fall. Many people mistakenly think that the shape of Earth’s orbit is what causes the seasons. However, the true cause of Earth’s seasons has more to do with its rotation than its revolution! Earth rotates on an axis that goes through the North and South Pole which is tilted 23.5° from Earth’s orbital plane. This means that each hemisphere is tilted toward the Sun for part of the year and away from the Sun for part of the year; and this is what causes Earth’s seasons. When one hemisphere is tilted toward the Sun, it receives direct sunlight resulting in warmer temperatures. At the same time, the other hemisphere is tilted away from the Sun and receives indirect sunlight. This means that the energy from the Sun is spread out over a larger area, reducing its intensity and resulting in cooler temperatures. When the Northern Hemisphere is tilted toward the Sun, we experience summer. When it is tilted away from the Sun, we experience winter.
Earth’s tilt also causes the number of hours of daylight to vary throughout the year. Earth rotates around its axis once every 24 hours, called a day. When the Northern Hemisphere is tilted toward the Sun, we are in the daylight longer (more than 12 hours of daylight). The longest day of the year, when we get the most direct sunlight, is called the summer solstice (@June 21). When the Northern Hemisphere is tilted away from the Sun, we are in the daylight for a shorter amount of time (less than 12 hours). The shortest day of the year, when we get the least direct sunlight, is called the winter solstice (@December 22). Of course, these days are the opposite for the Southern Hemisphere. Then, there are dates in between the summer and winter solstice where each hemisphere receives 12 hours of daylight and 12 hours of darkness. These are called the spring equinox (@March 20) and the fall equinox (@September 22). On these days, the most direct sunlight is over the Equator and both hemispheres experience moderate temperatures.
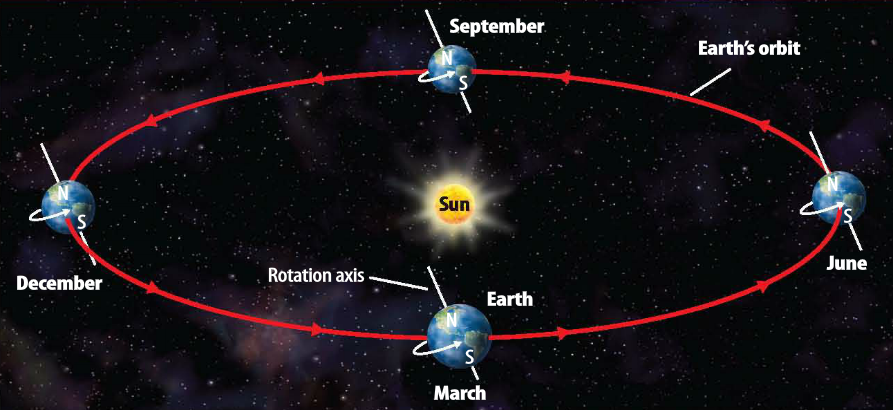
You may encounter diagrams similar to the one shown here that attempt to explain the seasons. However, as you know, models like this one can sometimes be misleading. The point of view is tilted to look at Earth’s orbit from an angle. This causes the nearly-circular ellipse of Earth’s actual orbit to look like an extremely long ellipse. This can give the reader a false impression of the shape of Earth’s orbit, which may play into misconceptions about the seasons as well.
EARTH AND THE MOON
Earth is also impacted greatly by its relationship with the Moon. While Earth orbits the Sun once per year, the Moon orbits Earth about 12 times per year, or once per month. Much like the Earth, the Moon also rotates as it revolves. However, the Moon’s rotation is much slower than Earth’s. Its period of rotation is about once per month, which is the same as its period of revolution. This means that the same side of the Moon is always facing the Earth.
Moon Phases
The Moon appears slightly different each night throughout the month, with different portions of the Moon’s surface being lit by sunlight. This is because its location relative to the Earth and the Sun changes as it revolves around the Earth. The lit part of the Moon that is visible from Earth is called a phase. When the moon is between the Earth and the Sun, the lit side of the Moon is facing away from Earth, so we do not see it. This is called a “new moon”. During the first two weeks of the month, when the lit part of the Moon visible from Earth gets larger, we say that the phases are “waxing”. When the Moon is on the opposite side of Earth from the Sun, the entire side facing the Earth is lit by sunlight. We call this a “full moon”. During the last two weeks of the month, the lit portion of the Moon that is visible from Earth gets smaller. We refer to these phases as “waning”.


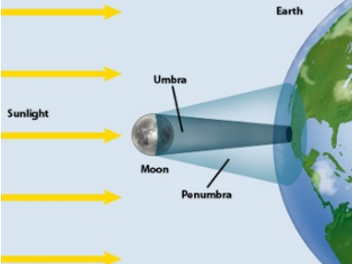
Eclipses
When the Earth and Moon line up with the Sun during their orbits, they cast a shadow on one another. This is called an eclipse. A solar eclipse can only happen during a new moon, when the Moon is between the Earth and the Sun. When this occurs, the Moon casts a shadow on Earth’s surface, blocking out the sunlight for that part of the Earth. A lunar eclipse can only happen during a full moon, when the Earth is between the Sun and the Moon. This causes the Earth to cast a shadow on the Moon.
However, eclipses do NOT occur twice each month during every new moon or full moon. This is because the Moon’s orbital plane is tilted from the Earth’s orbital plane by about 5°. If this weren’t the case, we would never see a full Moon! Because its orbit it tilted, the Moon only crosses the Earth’s orbital plane twice a month. In order for there to be an eclipse, the Moon must be in line with the Earth and Sun at the exact time that it crosses Earth’s orbital plane. This is why eclipses are so rare.
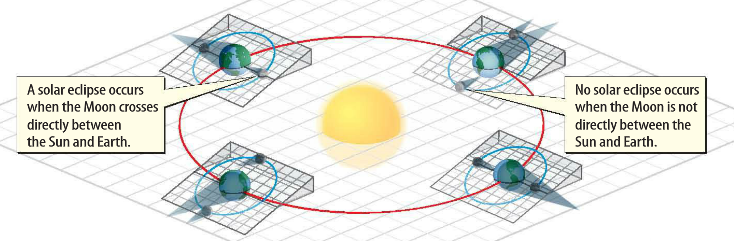
Tides
The relative positions of the Sun, Earth, and Moon also cause Earth’s ocean tides, the daily rise and fall of sea level. Because of the Moon’s gravitational pull, the water on Earth’s surface bulges on the side of Earth facing the Moon as well as the side of Earth opposite the Moon. This means that high tides occur when we are facing the moon or when we are on the opposite side of Earth from the Moon. When our location on Earth is perpendicular to the Moon, we experience low tides.

The Sun’s gravity can affect tides as well, though it is only about half as strong as the Moon’s tidal influence. During the full moon and new moon phases, when the Sun and Moon line up with the Earth, tides become stronger because the gravitational forces are in line with one another. This causes higher high tides and lower low tides, called spring tides. When the Moon is perpendicular to the Sun and Earth during the first quarter and third quarter phases, the Sun’s gravity reduces the effect of the Moon’s gravity on tides. This causes weaker tides, lower high tides and higher low tides, called neap tides.
THE SOLAR SYSTEM
The idea that our Sun is just a star, like most of the other little specks of light in the night sky, is a relatively new idea. Similarly, the idea that Earth is just one of several planets orbiting the Sun wasn’t widely accepted until fairly recently in human history. A star, like our Sun, and all of the objects that orbit around it make up what is referred to as a solar system; and ours is but one of countless solar systems in the universe. Scientists currently apply the nebular theory to explain how the solar system formed. The nebular theory states that stars form when massive clouds of dust and gas condense around points which become stars. Dust and gas surrounding the new star flattens into a disc and condenses into all of the other objects in the solar system, including the planets.
Historical Models
Prior to the 1600’s, most scholars believed that the Earth was the center of the whole universe. This theory is called geocentrism. The geocentric model states that all celestial objects, including the Moon, other planets, the Sun and stars, revolve around the Earth in concentric shells. However, mathematical models based on this theory never seemed to match actual observations of the motion of the planets. Beginning in the mid 1500’s and throughout the 1600’s, astronomers such as Copernicus, Tycho Brahe, and Galileo began to popularize a new way to think about planetary motion. Their theory, called heliocentrism, placed the Sun at the center of the solar system. In the heliocentric model, the planets (including Earth) all revolve around the Sun, which is one of countless stars in the universe. This model was better able to accurately predict the motion of planets as observed from Earth, and has been developed into our current understanding of our solar system.
Planets
Until recently, we included 9 planets in our model of the solar system. However, in 2006, the scientific definition of a planet changed so that Pluto, once the 9th planet from the Sun, was no longer considered a planet. Currently, we apply three criteria to determine if an object is a planet. First, it must orbit a star. Second, it must be massive enough to be rounded by its own gravity. Finally, the condition which stripped Pluto of its planetary status, it must be significantly more massive than objects in its orbital path. The eight planets in our solar system are divided into two distinct groups, the inner planets and the outer planets.
Inner Planets
The four planets closest to the Sun are often referred to as the inner planets. The inner planets include, in order from the Sun, Mercury, Venus, Earth, and Mars. These four planets are sometimes called “terrestrial” planets because they are mostly made of rocky material. The inner planets are much smaller than the outer planets and have fewer moons, warmer temperatures, and faster orbits. Mercury is the smallest planet as well as the closest to the Sun. Venus is similar in size to the Earth. It is the hottest planet despite the fact that Mercury is closer to the Sun. This is because Venus has a very dense atmosphere that traps the heat of the Sun on the planet’s surface. Earth, the third planet from the Sun, is the only planet of the eight in our solar system known to support life as a result of its moderate temperatures and protective atmosphere. The fourth of the inner planets, Mars, is about half the size of Earth and is known for its red color as a result of iron oxide (rust) on its surface.
Outer Planets
The four planets furthest from the Sun make up the outer planets. These planets are significantly bigger than the inner planets and tend to have many moons, cooler temperatures, and ring systems surrounding them. The fifth planet from the Sun is Jupiter. It is the largest planet in our solar system; its mass is more than twice that of all of the other planets combined. Saturn, the sixth planet, is the second-largest planet and is known for its large and complex ring system. Jupiter and Saturn are sometimes referred to as gas giants due to their gaseous composition. The seventh planet, Uranus, has a diameter 4 times larger than Earth and is unique in that its axis of rotation is very close to the orbital plane. This causes Uranus to appear as though it is rolling sideways through its orbit. Uranus is thought to be made of an icy slush of water, ammonia, and methane. Neptune, the farthest planet from the Sun, is similar in size and composition to Uranus. It is unique in that it was not discovered by observation, but by mathematical prediction based on irregularities in the orbit of Uranus. These two planets, Uranus and Neptune, are sometimes called ice giants because of their icy composition.

Dwarf Planets
After the criteria for classifying planets was changed in 2006, Pluto was reclassified as a dwarf planet. Dwarf planets are spherical objects that orbit the Sun, but they do not have a cleared orbital path. In other words, they orbit near other large objects. Many people don’t realize that there are several dwarf planets in our solar system in addition to Pluto, including one in the asteroid belt between Mars and Jupiter named, Ceres. Dwarf planets tend to be made of rock and ice, and are all smaller than Earth.
Moons
One might question what makes our Moon different from Pluto or Ceres. The answer is a key distinction between the two objects. While a planet or a dwarf planet orbits a star, a moon orbits a planet. We know that Earth has just one moon, but many of the planets have multiple moons. Jupiter, for example, has 67 known moons and Saturn has 62.
Asteroids & Comets
Small, rocky objects in our solar system that are not spherical and do not fit the criteria for planets and dwarf planets are known as asteroids. There are millions of asteroids that orbit the Sun in the asteroid belt between Mars and Jupiter and millions more that orbit the Sun in the region outside of the orbit of Neptune known as the
Kuiper belt.
While asteroids are rocky, there are also small objects orbiting the Sun that are made of gas, dust, and ice. These objects are known as comets. They tend to have extremely elliptical orbits that reach the far edges of the solar system, and scientists estimate that there may be more than a trillion comets orbiting our Sun.
STARS
When we look into the night sky, we can see countless stars shining brightly. These stars, like our Sun, are massive balls of gas held together by gravity with a core so hot and dense that nuclear fusion occurs. Stars are formed from immense clouds of dust and gas called nebulae. When areas of higher mass condense, the heat and pressure generated can begin the process of nuclear fusion, which gives birth to a new star.
Properties of Stars
With the help of early telescopes, Galileo was able to make observations about the Sun that had been impossible before. One interesting thing he noticed was that the Sun has spots, which we now know are regions of high magnetic activity. He observed that the sunspots move across the surface of the Sun, leading to the conclusion that the Sun rotates similarly to the Earth. One key difference, however, is that the plasma at the center seems to rotate faster than at the poles. In addition to sunspots, we also observe clouds of gas and bright eruptions from the surface of the Sun, called prominences and solar flares. Coronal Mass Ejections (CME’s) are giant bubbles of gas ejected from the Sun’s corona. The Sun is also constantly spewing charged particles into space. This stream of charged particles is known as the solar wind, and is responsible for the northern lights when it interacts with Earth’s magnetic field.
Stars are classified by their temperature, which determines the color they appear. The Sun, a yellow star, is a medium-temperature star. Blue stars, the hottest stars, tend to burn through their fuel the fastest, while the cooler red stars tend to burn through their fuel slower.
The absolute magnitude of a star refers to its actual brightness. However, because some stars are closer and some are farther away, their appearance may not accurately reflect their true brightness. This is why scientists use a scale called apparent magnitude to compare the brightness of all of the objects in the sky. The brightest object in the sky is, by far, the Sun. Surprisingly, the Moon and Venus are the second and third brightest objects based on apparent magnitude despite the fact that neither produces its own light but only reflects the Sun’s light. This is because they are much closer to us than any other objects.
When scientists compare the absolute magnitude of a star to its temperature, patterns begin to emerge. A chart called a Hertzsprung-Russell Diagram (H-R Diagram) shows these relationships. Most of the stars plotted on the H-R Diagram are part of what is known as the main sequence.
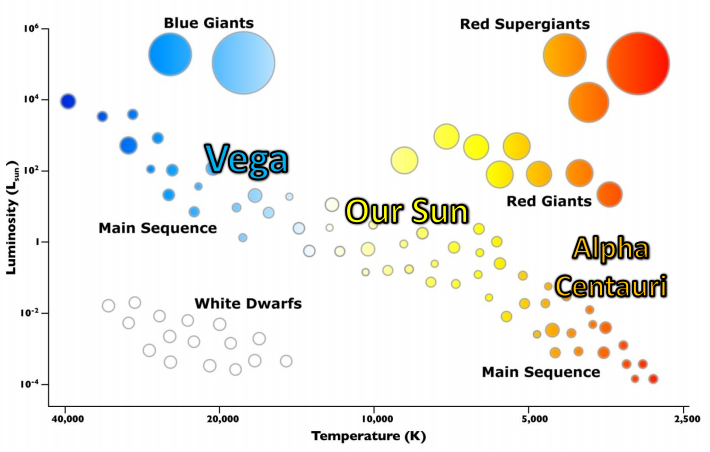
Stellar Life Cycles
When stars are born from condensing regions of a nebula, they begin fusing hydrogen atoms into helium, releasing massive amounts of energy in the form of heat and light. These stars, at the beginning of their life cycles, are called main sequence stars. When main sequence stars have used up most of their hydrogen, they collapse on themselves. This causes the helium atoms to begin fusing into carbon. Stars in this phase of their life cycle are known as red giants.
In lower-mass stars, when the helium runs out, they cannot get hot enough to fuse carbon atoms and nuclear fusion stops. The remnants of these stars are called white dwarfs, as the remaining carbon is so hot that it continues to glow. Over time, the white dwarf will slowly cool down until it is just a cold, black sphere. These are called black dwarfs, and they signal the end of the life cycle of a low-mass star.
When stars of higher-mass collapse at the end of the red giant stage, they become red supergiants that can fuse heavier and heavier elements up to iron. Once they run out of fuel at this stage, they collapse and explode in what is known as a supernova. A supernova results in one of two outcomes. Most of these exploding stars become what is called a neutron star, which is a dense core of neutrons that remains after the supernova that spin rapidly as they emit bright beams of light. The heaviest of stars will collapse again into a sphere so massive that its gravity will prevent even light from escaping. This is known as a black hole. It is difficult to observe a black hole because it does not reflect light, however, we can study them by observing how they affect nearby objects.
THE UNIVERSE
Distances
We use words such as “nearby” pretty loosely when we refer to objects in space. The distances between objects are almost inconceivable for us. Even the distances between planets would stretch our ability to understand if we used standard measurements such as kilometers or miles, so astronomers decided to invent new units of measure that are more friendly to the incredible distances between objects in our universe.
Within our solar system, scientists use astronomical units (AU) to describe the distances between objects. One astronomical unit is equal to the average distance between the Earth and the Sun, which is about 150 million kilometers. For reference, Earth is a distance of 1 AU from the Sun. Because Mars is a distance of 1.52 AU from the Sun, this means that Mars’ orbit is about 50% further from the Sun than Earth’s. Neptune, the furthest planet from the Sun, is a distance of 30.1 AU. Proxima Centauri, the nearest Star to our solar system, is a distance of 268,000 AU from the Sun.
As you can imagine, if the nearest star is that far away, scientists cannot rely on AU to measure distances beyond our solar system. That is why they use another unit called a light year; which, despite its name, measures distance rather than time. A light year is the distance that light can travel in one Earth year. For reference, the distance between Earth and the Sun is 8 light-minutes (it takes light 8 minutes to travel 1 AU). The distance between our Sun and Proxima Centauri is about 4.3 light years. The distance from our Sun to the opposite side of our galaxy, the Milky Way, is about 52,000 light years. This means that when we look at a star on the far side of the galaxy, we are seeing light that has been travelling through space for 52,000 years. In other words, we are seeing what that star looked like 52,000 years ago!
Solar Systems, Galaxies, and Galaxy Clusters
Astronomers have been able to observe planets orbiting other stars than our Sun. It is conceivable that most, if not all of the stars in our universe have some kind of planetary system orbiting around them. Considering that there are countless stars in our observable universe, it’s a good possibility that there are countless solar systems with many countless numbers of planets as well.
Sometimes, stars travel so close to each other that they become bound by each other’s gravity. Such stars orbit each other in what is known as a binary star system. Stars also tend to clump together in relatively close proximity to each other in what is known as a star cluster. These clusters are part of a larger system of hundreds of billions of stars grouped together by gravity called galaxies.
There are several types of galaxies, including spiral galaxies such as our own Milky Way. A spiral galaxy is shaped much like a hurricane, with billions of stars spinning in spiral arms around a central point that some scientists believe may be a black hole. Our Sun is located near the end of one such spiral arm of the Milky Way, called the Orion Arm. There are also elliptical galaxies and irregular galaxies. However, some scientists theorize that elliptical galaxies are simply spiral galaxies viewed from the “side” and that irregular galaxies are multiple galaxies that collided and are “mixing” together. It’s interesting to think of galaxies colliding with each other, and easy to imagine millions of stars smashing into one another. However, the distances between stars is so great that even when galaxies collide, stars rarely come close enough to each other to “crash” together.
Expansion
Astronomers who observe distant galaxies have recorded an interesting phenomenon that all galaxies are moving away from us, and from each other for that matter. This means that the universe must be expanding. Imagine marking dots on the surface of a deflated balloon. If you were to begin blowing up the balloon, all of the dots would be getting further apart from one another. This observed “expansion” of the universe is one of the key pieces of evidence supporting the theory that the universe originated from a central spot, known as the “big bang theory”.
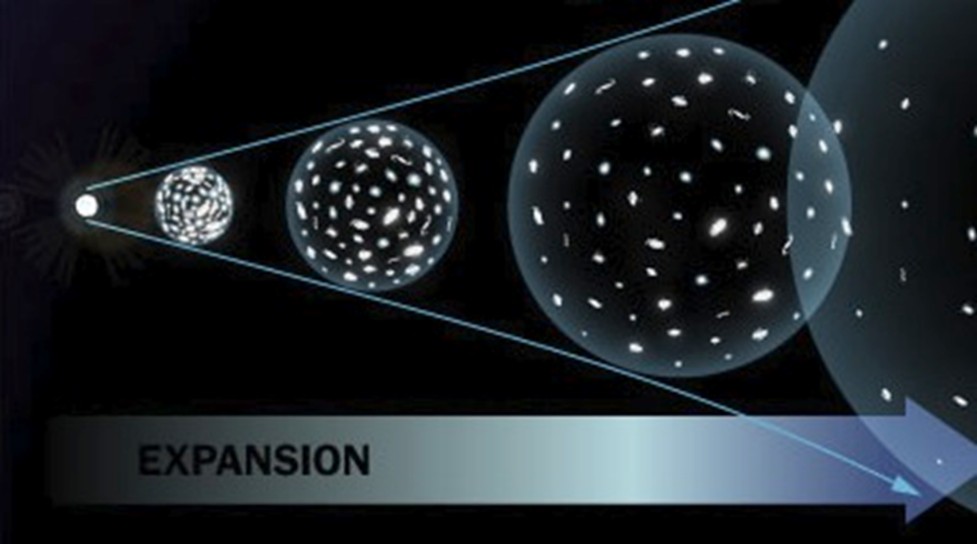
Коментари